Weaving machine manufacturers have introduced numerous significant advances in weaving and weaving preparation. Advances include individual spindle drives in winding; faster sample warpers permitting a range of colors; a high level of automation in indirect warping; faster and smarter drawing-in and tying-in; quick style changes; automatic pattern changes; powerful weaving machine drives; variable pick density; variable loom speed; adaptive control systems for management of main and auxiliary air nozzles timing to reduce energy in air-jet weaving; on-loom monitoring of woven fabric quality; and individual control of harnesses and warp yarns in dobby and jacquard weaving, among countless other innovations. These developments permit high-speed fabric formation with improved fabric quality and versatility.
Innovations In Dobby Shedding Systems
Dobby shedding motion controls the movement of large number of harnesses. As the number of harnesses increases, so does the load on the motor that drives all moving parts connected to the weaving essential motions — shedding, weft insertion, beat up, and warp and fabric controls — and the weaving secondary motions — warp stop, weft stop, weft selection, leno and tuck-in. When the weaving process is stopped for any reason —warp break, weft break, or other stop — the potential for start-mark defects exists. When weaving is resumed, the motor initially has to overcome the inertia of the machine’s heavy moving parts, and the machine’s set speed is not reached immediately. Consequently, the beat-up force is weaker and the new pick is not forced far enough and stays slightly further from the cloth fell creating a start-mark defect. Innovations developed by weaving machine manufacturers and shedding systems producers offer solutions for start-mark defects as well as other benefits.
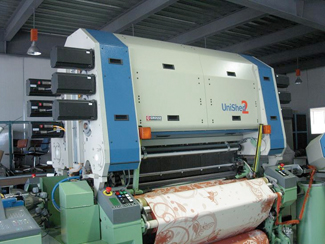
Figure 1: The commercial version of Grosse’s Unished 2 mounted directly on a weaving machine frame
Using a single drive for the entire weaving machine, Germany-based Lindauer Dornier was the first to pursue a system that can eliminate start-mark defects. The company exhibited its SyncroDrive system for the first time at ITMA 2007 in Munich, Germany. The drive focuses on the stability of motor rotational speed. SyncroDrive separates the large dobby mass from the drive at the starting moment, and then engages the dobby shedding system after the inertia of other moving parts has been overcome. This permits the weaving machine to reach the set weaving speed during the first weaving cycle after the start and before beat-up, thus eliminating start marks.
Switzerland-based Stäubli was the first company to pursue the development of a dobby system with individual control of each harness. The UNIVAL 500 — which uses powerful actuators, one for each harness, to control harness movement — was an expansion of the company’s Unival 100 concept. The actuator’s direction of rotation determines which harnesses are raised or lowered to form the shed according to the weave design.
At ITMA 2011 in Barcelona, Spain, Toyota Industries Corp., Japan, joined the competition for individual harness control of dobby shedding systems. The company named its system “E-She” for electronic shedding system. Germany-based Mageba Textilmaschinen GmbH & Co. KG also exhibited its narrow-shuttle weaving machine SL-MT 1/180 combined with a dobby shedding system. In this shedding system, a separate motor drives each harness. Thus, each harness can be raised or lowered at different levels to form multi-sheds and allow for multi-weft insertion.
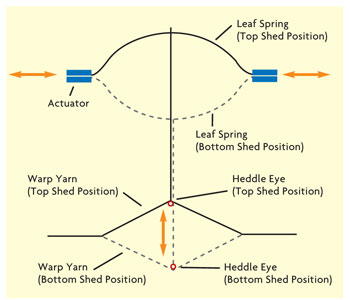
Figure 2: Illustration showing Unished’s shed formation principle
Benefits Of Individual Harness Control
The individual harness drive allows easy shed timing and independency of shed timing from other events, while synchronizing all events to work in harmony during a given weaving cycle. The shed timing in a traditional shedding motion is rigid and fixed for each weaving cycle. The individual control of harnesses makes it possible to time the shed differently for each weaving cycle if desired. For instance, in weaving fabrics with a mixture of weft yarns — some containing spandex and others without — the shed timing for the former requires early shed to bind these yarns before the beat-up event so they are secured and bound to prevent the spandex yarn from retracting back and causing major fabric defects. With such innovation, high-stretch spandex yarns easily can be woven. In general, early shed is not desired because of the dragging force of the weft in crossed warp yarns, which causes abrasion and weakening of the warp and weft yarns. Yarns without spandex can be timed in a normal shed whereby warp yarns are leveled at the beat-up event. The combination of early shed and normal time shed is useful when weaving variable pick density where one or more of the pick densities are set to a maximum and the other densities are at lower values than the maximum. Early shed is required for the maximum pick density stripes.
In traditional dobby systems, there is an electromagnet and several links for each harness. In dobby shedding with individual harness control, the electromagnets and links are eliminated. Also, the load on the main motor that drives the other weaving mechanisms is reduced. This combination allows significant noise and vibration reduction as well as increases in weaving speed.
One of the reasons for limiting the number of harnesses in traditional dobby was concern for significant load on the motor, which would limit design capability. This is not an issue with individual harness control. Stäubli offers its Unival 500 in more than 50 harnesses. With such high number of harnesses, intricate dobby designs, which were not possible before, can be created. Because each harness can be raised or lowered at different heights for each pick if needed, formation of weaves with longer floats before the next intersection can be produced. The height of a harness can be controlled to absorb the slackness of the floated yarn or yarns until the weave intersection takes the extra length of floated yarns. Again, this is a plus in terms of design intricacy.
In terry weaving, the shed configuration requires setting the harnesses that control the pile yarns differently than standard fabric settings. The pile harnesses must be raised higher than the upper shed of the ground yarns and lower than the bottom shed of the ground yarns. Such settings require mechanical adjustment of the pile harnesses in traditional dobby shedding. In the case of individual harness control, this can be done automatically via the controller, typically a touch-screen interface, of the pile harnesses.
Innovations In Jacquard Shedding Systems
Until the 1980s, the mechanical jacquard systems were most commonly found in industrial settings and they still exist today in developing countries. These systems have too many parts: cylinder, pattern cards, needles, needle springs, hooks, neck cords, harness cords, heddle wires, and returning springs for each harness cord. The parts work in harmony for selection of hooks, and hence warp yarns, to be raised or lowered for each shed according to the weave design that is registered on the punch pattern cards. The jacquard head parts get their movement from a drive connected to the main weaving machine motor, which adds more complications because each weaving machine has a different main drive configuration requiring different connection configuration to the jacquard head. The high number of mechanical parts for jacquard machines along with their heavy mass and complicated motions limited weaving speed to a degree that jacquard was not compatible with shuttleless high-speed weaving.
Traditional jacquard capacity, whether mechanical or electronic, was limited, which requires harness tie and weaving several small to medium design repeats across the fabric width. This posed challenges for design innovation and flexibility. The standard size or capacity of jacquard machines ranges from 448 to 1,792 hooks. A given harness tie type — straight, center, border, or mixed harness tie — has remained unchanged for a long time because their construction takes a fairly long time. For this reason, a jacquard designer has to fit patterns to existing harness ties. Jacquard machine manufacturers responded to the design capability challenge by introducing individual control of harness cords (or warp yarns), and mega capacity. In mega-sized jacquard machines offered by Stäubli, the number of hooks may reach up to 18,432. The individual warp yarn control and mega-sized jacquard machines permit the creation of extremely intricate large-sized patterns, one large pattern across the entire fabric width, or several small- to medium-sized patterns across the fabric width may be created.
Grosse’s UNISHED
The earliest version of Germany-based Grosse’s UNISHED was exhibited at ITMA 1999 in Paris as a prototype. The machine, known as Unished 1, was shown again at ITMA 2003 in Birmingham, England, with little improvement. At ITMA 2007, the much improved Unished 2 was exhibited, and approximately two years later the machine was available commercially. Figure 1 shows the commercial version of the Unished 2 mounted directly on the weaving machine frame weaving one large pattern across the entire fabric width. Figure 2 illustrates the shed formation principle of the Unished. The configuration of the Unished led to the elimination of harness cords, magnets, hooks, pulleys, springs, and the gantry. At ITMA 2011, the Unished 2 was shown on the Tsudakoma booth installed on a water-jet weaving machine, the ZW8100. This was the first time a water-jet weft-insertion weaving machine was combined with a jacquard shedding system at ITMA.
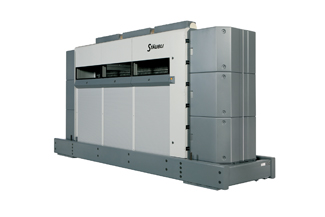
Figure 3: The commercially available version of Stäubli’s Unival 100.
Stäubli’s UNIVAL 100
At ITMA 2003, Stäubli introduced the Unival 100 in its commercial configuration (See Figure 3). Figure 4 illustrates how the shed is formed. In the figure on the left, an actuator — an extremely small stepping motor Stäubli named JACTUATOR — is shown while selecting its harness cord, the warp yarn, for the upper shed. The image on the right shows a jactuator with its warp yarn selected for the bottom shed. In this system, magnets, hooks, knives, and pulleys are eliminated. At ITMA 2003, a Unival 100 with capacity of 7,920 was mounted on a Picanol OMNIplus-6-J 250 weaving machine and demonstrated weaving mattress ticking and table cloth fabrics where the pattern was changed on the fly at 1,025 picks per minute and a filling insertion rate 2,460. This rate of filling insertion is the highest in the history of jacquard weaving. With this technology, weavers can manufacture intricate jacquard designs at the speed of commodity fabrics. The configuration of the Unival 100 is modular, which allows the capacity to vary from an extremely small number of cords for narrow fabrics and label weaving, to extremely large numbers for broad weaving. The Unival 100 is available in a variable capacity of up to 20,480 jactuators.
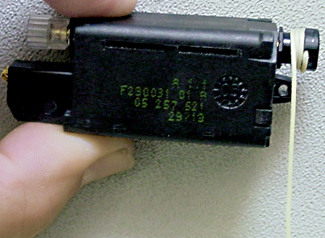
Figure 4: Top shows Stäubli’s Jactuator — a feature on the Unival 100 — while selecting a warp yarn for upper shed; below shows the Jactuator while selecting a warp yarn for bottom shed.
With the Unival 100, the shed can be optimized for each filling insertion, depending on the quality and type of yarn used.
Because there are no knives that raise the yarns at the same shed height, warp yarns may be raised to different heights. The Unival offers true individual warp yarn control. This feature provides major opportunities for weavers. With such a system, several sheds can be formed allowing the weaving of face-to-face pile fabrics and 3-D structures including 3-D orthogonal preforms for composite applications.
Opportunities
Historically, dobby and jacquard woven fabrics have offered countless colors and intricate textured designs, and as such, have established themselves in commercial applications such as high fashion dresses, decorative fabrics, drapery, sheeting, upholstery, matelassé fabrics, mattresses, tablecovers, wall décor, terry cloth and woven carpets. Jacquard weaving also extended to non-traditional technical textile applications such as seamless airbags and awnings. Other potential applications that are still undergoing research and development include electronic textiles, shaped seamless apparel, and 3-D shaped preforms for fiber-reinforced composites. The new innovations in shedding systems may help realize these potential applications.
3-D Woven Structures
One potential application of individual warp yarn/harness control is 3-D orthogonal and other 3-D woven fabrics for numerous markets including fiber reinforced composites for aerospace, automotive, boats, bridges, armor and wind energy blades end-uses. In 3-D orthogonal weaving, multi-sheds are formed for multi-weft insertion. Figure 5 shows a 3-D weaving machine located at the Composite Core Facility, College of Textiles, North Carolina State University (NCSU), United States, donated by 3TEX Inc., United States. The figure shows the machine weaving a preform comprised of four warp layers and five weft layers. In 3-D orthogonal weaving, the weft yarns are inserted simultaneously using five rapiers. The top and bottom layers are the binding yarns and the four layers in the middle are the warp yarns. The binding yarns’ movements are controlled by harnesses, while the warp yarns are stationary and as such do not require harnesses, but merely are threaded through guides to situate them as seen in the figure. Figure 6 shows an example of a 3-D orthogonal structure with seven warp layers, eight weft layers and binding yarns in a plain weave structure. The machine shown in Figure 5 is dedicated to weaving 3-D orthogonal woven structures. Individual warp yarn/harness control shedding systems with all yarns controlled by actuators/harnesses can be employed to form 3-D orthogonal structures and weave other 3-D fabrics such as interlocks, angle stitched, and cellular structures woven individually or combination of these in the same fabric, which provides design flexibility for different applications.

Figure 5: A 3-D weaving machine producing a preform fabric featuring four warp layers and five weft layers
Multiphase Weaving
The main target of multiphase weaving is to overcome the weaving speed limitation of traditional single-phase weaving. In single-phase weaving, one pick is inserted at a time, while multiple filling insertions take place simultaneously in the case of multiphase weaving. The first commercially viable multiphase weaving machine was unveiled at ITMA 1995 by the then Sulzer Textil, Switzerland. The M8300 was well received by the weaving industry, and was one of the main attractions at ITMA 1995, 1999, and 2003 because of its high filling insertion rate — about three time as fast as the rate for a single-phase machine. However, the machine’s limitations resulting from the way the warp yarns are manipulated to form sheds and the beat-up of weft yarns — including fixed warp density; low and medium range warp density; limited weave design; and low to medium pick density range — were quickly realized. The M8300 was ultimately discontinued because of low demand.
However, there is a demand for multiphase weaving machines that do not possess the limitations of the M8300. Dobby and jacquard shedding systems with individual harnesses/warp yarn controls provide the mechanism to form multi-sheds. These shedding systems combined with elements of the M8300 could potentially lead to the development of multiphase weaving machines with the ability to create dobby and jacquard woven designs for home furnishing, fashion, and industrial markets. Needless to say, this requires a joint venture between weaving machine manufacturers and dobby and jacquard shedding systems producers.

Figure 6: An illustration of a 3-D orthogonal woven structure featuring 7 yellow warp layers, 8 red weft layers and green plain-woven binding yarns.
Electronic Woven Structures
Electronic textiles, also known as electrotextiles or e-textiles for short, are textile structures containing electrically conductive yarns, electronic sensors, optical fiber sensors, actuators or a power source. These electronics are interconnected via a circuit to provide functionality to the e-textile and hence form a smart system that can sense, respond and adjust to stimuli. E-textile products are available in many commercial applications such as thermal clothing to protect from cold weather; musical jackets; interactive drawing pads; and flexible, foldable computer keyboards. Numerous potential defense and civilian applications of e-textiles have been identified and researched including environmental sensors; global positioning systems; imaging using small cameras; antennas; acoustic arrays that identify a sound source such as gun shots and enemy vehicles; formation of transistors on flexible substrates such as thin ribbon yarns; and yarn batteries and flexible and conformable solar cells to harvest and provide electrical power to the e-textiles.
Currently, e-textiles are produced by weaving or knitting fabrics using electrically conductive yarns. Then a series of manual processes forms electronic circuit interconnects and embeds and connects sensors and power sources within the e-textile. The technology’s potential for growth is challenged by these manual processes that limit productivity and increase cost, which is a serious impediment for commercialization.
Jacquard weaving machines with individual warp-yarn control offer the greatest potential for producing complete systems of e-textiles automatically at high-speed right at the weaving machine with minimal or no manual processes following. In jacquard weaving, every warp and filling yarn and their cross-over points are addressed, which would allow robotic guided resistive or ultrasound welding devices to identify the welding points and form interconnects using welds to create a woven circuit. Integrating sensors — say a microphone, for example — to the woven circuit requires conductive yarns with weave float, which can be incorporated into the woven design using computer-aided design. Dispensing buttons and connecting them to clothing automatically has long been known to the apparel industry. Sensors, in the form of buttons, can be developed and attached to the woven circuit using conductive yarns to connect the sensors.
The future holds promises of electronic elements in yarn form including battery yarns, solar cell yarns, and ribbon yarns with built-in electronic devices. Jacquard weaving technology needs to be augmented with supporting devices still to be developed, such as filling yarn feeders that can handle optic fibers, yarn batteries and ribbons without twisting them; and creels behind the machine to feed electronic yarns in the warp direction. Serious collaboration between the electronics industry, woven fabric manufacturers, the apparel industry and weaving machine producers is a must to make this happen.
Automatic Warp-Break Repair
While today’s weaving machines are highly automated and equipped with electronic systems that stop the loom when a filling or warp yarn breaks, changing woven design on the fly, repairing broken filling yarns or varying pick density, for example, locating and repairing a broken warp yarn still requires operator intervention. The opportunity for locating a broken warp yarn seems to be within reach using jacquard weaving with individual warp yarn control. Research conducted at NCSU’s College of Textiles and funded by the United States-based National Textile Center demonstrated the concept by instrumenting selected harness cords with a micro-electro-mechanical (MEMS)-based accelerometer. In this system, the harness cord vibrates differently when in the presence of the warp yarn versus when it is broken, and the difference can be sensed by the MEMS accelerometer. Since the harness cord is addressed along with its warp yarn, the break location can be identified a manner that saves on repair time and increases efficiency. It is envisioned that the jacquard head combined with a robotic system and yarn splicing will be able to repair the broken warp yarns. The concept of using a MEMS accelerometer can be used by any jacquard system using harness cords. The Unival 100 Jactuator from Stäubli, however, can be programmed to sense and identify warp breaks locations by knowing the load on the jactuator in the presence of the yarn and when it is broken without the need for additional sensing such as MEMS accelerometer.
Editor’s Note: Dr. Abdel-Fattah M. Seyam is a professor at North Carolina State University’s College of Textiles, Department of Textile and Apparel Management, United States. This article is based on Seyam’s presentation given at the 2014 Textile World Innovation Forum.
April/May/June 2015